Abstract
Although automation has advanced in manufacturing, the growth of automation in construction has been slow. Conventional methods of manufacturing automation do not lend themselves to construction of large structures with internal features. This may explain the slow rate of growth in construction automation. Contour Crafting (CC) is a recent layered fabrication technology that has a great potential in automated construction of whole structures as well as sub-components. Using this process, a single house or a colony of houses, each with possibly a different design, may be automatically constructed in a single run, imbedded in each house all the conduits for electrical, plumbing and air-conditioning. Our research also addresses the application of CC in building habitats on other planets. CC will most probably be one of the very few feasible approaches for building structures on other planets, such as Moon and Mars, which are being targeted for human colonization before the end of the new century. Contour Crafting is an emerging technology that uses robotics to construct free form building structures by repeatedly laying down layers of material such as concrete. The Contour Crafting technology scales up automated additive fabrication from building small industrial parts to constructing buildings. Optimal machine operation planning for Contour Crafting benefits the technology by increasing the efficiency of construction, especially for complicated structures. The research reported here has aimed at providing a systematic solution for improving the overall Contour Crafting system efficiency in building custom-designed buildings. An approach is first presented to find the optimal machine operation plan for the single nozzle Contour Crafting system. Other approaches are then presented to determine collision-free operation plans for machines with multiple nozzles. The models developed incorporate physical constraints as well as some practical construction issues.
Although automation has advanced in manufacturing, the growth of automation in construction has been slow. Conventional methods of manufacturing automation do not lend themselves to construction of large structures with internal features. This may explain the slow rate of growth in construction automation. Contour Crafting (CC) is a recent layered fabrication technology that has a great potential in automated construction of whole structures as well as sub-components. Using this process, a single house or a colony of houses, each with possibly a different design, may be automatically constructed in a single run, imbedded in each house all the conduits for electrical, plumbing and air-conditioning. Our research also addresses the application of CC in building habitats on other planets. CC will most probably be one of the very few feasible approaches for building structures on other planets, such as Moon and Mars, which are being targeted for human colonization before the end of the new century. Contour Crafting is an emerging technology that uses robotics to construct free form building structures by repeatedly laying down layers of material such as concrete. The Contour Crafting technology scales up automated additive fabrication from building small industrial parts to constructing buildings. Optimal machine operation planning for Contour Crafting benefits the technology by increasing the efficiency of construction, especially for complicated structures. The research reported here has aimed at providing a systematic solution for improving the overall Contour Crafting system efficiency in building custom-designed buildings. An approach is first presented to find the optimal machine operation plan for the single nozzle Contour Crafting system. Other approaches are then presented to determine collision-free operation plans for machines with multiple nozzles. The models developed incorporate physical constraints as well as some practical construction issues.
1. INTRODUCTION
1.1. General
Since the early years of the twentieth century automation has grown and prevailed in almost all production domains other than construction of civil structures. Implementation of automation in the construction domain has been slow due to: a) unsuitability of the available automated fabrication technologies for large scale products, b) conventional design approaches that are not suitable for automation, c) significantly smaller ratio of production quantity/type of final products as compared with other industries, d) limitations in the materials that could be employed by an automated system, e) economic unattractiveness of expensive automated equipment, and f) managerial issues. On the other hand, the following are reported to be serious problems that the construction industry is facing today:
• Labor efficiency is alarmingly low,
• Accident rate at construction sites is high,
• Work quality is low, and
• Control of the construction site is insufficient and difficult, and skilled workforce is vanishing.
Automation of various parts and products has evolved considerably in the last two centuries but with the exception of a few successful attempts (see for example Balaguer et al, 2002) construction of whole structures remains largely as a manual practice. This is because the various conventional methods of manufacturing automation do not lend themselves to construction of large structures. A promising new automation approach is layered fabrication, generally known as Rapid Prototyping (RP) or Solid Free Form Fabrication (SFF). Although several methods of rapid prototyping have been developed in the last 2 two decades (Pegna, 1997), and successful applications of these methods have been reported in a large variety of domains (including industrial tooling, medical, toy making, etc.), most current layered fabrication methods are limited by their ability to deliver a wide variety of materials applicable to construction. Additionally, they are severely constrained by the low rates of material deposition which makes them attractive only to the fabrication of small industrial parts. Currently Contour Crafting (CC) seems to be the only layer fabrication technology that is uniquely applicable to construction of large structures such as houses (Khoshnevis, 1998).
1.2. The Contour crafting technology:
Contour crafting can automatically construct custom-designed structures by repeatedly layering down construction material (Fig.1.1). Contour Crafting (CC) is an additive fabrication technology that uses computer control to exploit the superior surface-forming capability of toweling in order to create smooth and accurate planar and free form surfaces out of extruded materials. Some of the important aspects of CC are lower construction cost, superior construction speed, flexibility of architectural design, safety and friendliness to the environment. Extensive experiments have been conducted over the last few years to configure the CC process to produce a variety of small and full scale objects.
Contour crafting can automatically construct custom-designed structures by repeatedly layering down construction material (Fig.1.1). Contour Crafting (CC) is an additive fabrication technology that uses computer control to exploit the superior surface-forming capability of toweling in order to create smooth and accurate planar and free form surfaces out of extruded materials. Some of the important aspects of CC are lower construction cost, superior construction speed, flexibility of architectural design, safety and friendliness to the environment. Extensive experiments have been conducted over the last few years to configure the CC process to produce a variety of small and full scale objects.
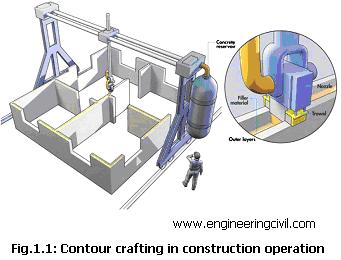
Small and medium sized 2.5D and 3D parts with square, convex, and concave curve features have been fabricated from a variety of thermoplastic and ceramic materials.
Larger Contour Crafting have been developed to build larger structures. These machines combine an extrusion process for forming the object surfaces and a filling process (by pouring, or extrusion) to build the object core. Several wall specimens have been constructed using CC machines that can produce hollow walls with corrugated internal structur .This design is expected to be a good initial candidate for concrete wall construction as is in a way equivalent to the current CMU based walls which are widely used in various construction applications. The CC machines are light weight and can be quickly assembled, disassembled and transported by a small crew. The construction operation can be fully automated requiring minimum supervision.
1.3. Process planning and optimization in Contour Crafting:
Operation planning and optimization play important roles in improving the overall CC system efficiency by generating optimal nozzle/trowel paths for the given structure designs. Multiple-nozzle or multiple-gantry systems would be suitable for construction of larger community and multi-residence structures to reduce the construction time and cost. Two types of Multi-Machine CC systems are considered in this study:
Operation planning and optimization play important roles in improving the overall CC system efficiency by generating optimal nozzle/trowel paths for the given structure designs. Multiple-nozzle or multiple-gantry systems would be suitable for construction of larger community and multi-residence structures to reduce the construction time and cost. Two types of Multi-Machine CC systems are considered in this study:
Overhead multi-nozzle and multi-gantry. In both cases specific schedule and workload are to be assigned to individual nozzles or gantries for collaborative operation. Collision between nozzles should be avoided without significantly compromising the overall constructing efficiency.
2. Contour Crafting:
Contour Crafting (CC) is an additive fabrication technology that uses computer control to exploit the superior surface-forming capability of troweling to create smooth and accurate planar and free-form surfaces. Some of the important advantages of CC compared with other layered fabrication processes are better surface quality, higher fabrication speed, and a wider choice of materials. The key feature of CC is the use of two trowels, which in effect act as two solid planar surfaces, to create surfaces on the object being fabricated that are exceptionally smooth and accurate. Artists and craftsmen have effectively used simple tools such as trowels, blades, sculpturing knives, and putty knives, with one or two planar surfaces for forming materials in paste form since ancient times. Their versatility and effectiveness for fabricating complex free-form as well as planar surfaces is evidenced by ancient ceramic containers and sculptures with intricate or complex surface geometries as well as detailed plaster work that have shapes as complicated as flowers, on the walls of rooms. Surface shaping knives are used today for industrial model making (e.g., for building clay models of car bodies). However, despite the progress in process mechanization with computer numerical control and robotics, the method of using these simple but powerful tools is still manual, and their use is limited to model building and plaster work in construction.
Contour Crafting (CC) is an additive fabrication technology that uses computer control to exploit the superior surface-forming capability of troweling to create smooth and accurate planar and free-form surfaces. Some of the important advantages of CC compared with other layered fabrication processes are better surface quality, higher fabrication speed, and a wider choice of materials. The key feature of CC is the use of two trowels, which in effect act as two solid planar surfaces, to create surfaces on the object being fabricated that are exceptionally smooth and accurate. Artists and craftsmen have effectively used simple tools such as trowels, blades, sculpturing knives, and putty knives, with one or two planar surfaces for forming materials in paste form since ancient times. Their versatility and effectiveness for fabricating complex free-form as well as planar surfaces is evidenced by ancient ceramic containers and sculptures with intricate or complex surface geometries as well as detailed plaster work that have shapes as complicated as flowers, on the walls of rooms. Surface shaping knives are used today for industrial model making (e.g., for building clay models of car bodies). However, despite the progress in process mechanization with computer numerical control and robotics, the method of using these simple but powerful tools is still manual, and their use is limited to model building and plaster work in construction.
In CC, computer control is used to take advantage of the superior surface forming capability of troweling to create smooth and accurate, planar and free-form surfaces. The layering approach enables the creation of various surface shapes using fewer different troweling tools than in traditional plaster handwork and sculpting. It is a hybrid method that combines an extrusion process for forming the object surfaces and a filling process (pouring or injection) to build the object core. The extrusion nozzle has a top and a side trowel. As the material is extruded, the traversal of the trowels creates smooth outer and top surfaces on the layer. The side trowel can be deflected to create non-orthogonal surfaces. The extrusion process builds only the outside edges (rims) of each layer of the object. After complete extrusion of each closed section of a given layer, if needed filler material such as concrete can be poured to fill the area defined by the extruded rims.
2.1. Objectives:
The reported work has aimed at the development and verification of a systematic methodology for process planning and optimization for most efficient construction of complicated large-scale structures by Contour Crafting for single and multiple machine scenarios through the following steps:
1. Describe system characteristics and define tool path elements of Contour Crafting
2. Develop practical tool path planning and an optimization method for the single nozzle CC system
3. Develop practical tool path planning and optimization methods for multi-nozzle systems based on the optimization method for the single nozzle case.
The reported work has aimed at the development and verification of a systematic methodology for process planning and optimization for most efficient construction of complicated large-scale structures by Contour Crafting for single and multiple machine scenarios through the following steps:
1. Describe system characteristics and define tool path elements of Contour Crafting
2. Develop practical tool path planning and an optimization method for the single nozzle CC system
3. Develop practical tool path planning and optimization methods for multi-nozzle systems based on the optimization method for the single nozzle case.
2.2. Operational facts:
The scale of the optimization problem may be too large when all aspects of the technology are considered. Operational facts should be established according to the limitation and features of the system. The following are the operational facts for the system:
1. The focus here is on finding the optimal tool path for 2.5 D structures. Layers are similar to each other except at the points where openings for windows or doors are located. Generally the nozzle will try to start and end at the same point in each layer.
2. In the construction process a nozzle or nozzles have to completely finish a layer before moving on to the next layer.
3. For each layer the CC nozzle has to finish depositing one wall segment completely before starting a new wall segment so that it will travel only between end points of each wall segments.
4. In order to avoid collision between the nozzle and the previously deposited walls the nozzle should be lifted up (by at least one layer height) when traveling between end points.
5. The nozzle will be idle while traversing the places where windows or doors are located. This traversal time is called “air time”.
6. The deposition flow rate can be perfectly controlled (i.e., concrete flow can start and stop) at any time.
7. Acceleration and deceleration times of the system are considered as fixed delays in the optimization analysis.
8. The focus is on developing a practical optimization method to generate tool paths for general structures. The maximum vertex number in a structure layout would be less than 10,000. This allows for handling fairly large and complex structures.
9. In the multi-nozzle case the nozzles always work on the same layer. It is assumed that allowing the nozzles to work on different layers at the same time will not increase the system efficiency.
The scale of the optimization problem may be too large when all aspects of the technology are considered. Operational facts should be established according to the limitation and features of the system. The following are the operational facts for the system:
1. The focus here is on finding the optimal tool path for 2.5 D structures. Layers are similar to each other except at the points where openings for windows or doors are located. Generally the nozzle will try to start and end at the same point in each layer.
2. In the construction process a nozzle or nozzles have to completely finish a layer before moving on to the next layer.
3. For each layer the CC nozzle has to finish depositing one wall segment completely before starting a new wall segment so that it will travel only between end points of each wall segments.
4. In order to avoid collision between the nozzle and the previously deposited walls the nozzle should be lifted up (by at least one layer height) when traveling between end points.
5. The nozzle will be idle while traversing the places where windows or doors are located. This traversal time is called “air time”.
6. The deposition flow rate can be perfectly controlled (i.e., concrete flow can start and stop) at any time.
7. Acceleration and deceleration times of the system are considered as fixed delays in the optimization analysis.
8. The focus is on developing a practical optimization method to generate tool paths for general structures. The maximum vertex number in a structure layout would be less than 10,000. This allows for handling fairly large and complex structures.
9. In the multi-nozzle case the nozzles always work on the same layer. It is assumed that allowing the nozzles to work on different layers at the same time will not increase the system efficiency.
2.3. Tool path definition:
Contour Crafting can save considerable time and cost as compared with the traditional way of construction. The cost of construction is related to time and energies spent by the machine and the amount of materials consumed for the structure. The total construction can be evaluated once a tool path has been defined. The building model is first sliced into layers, and then the layout of one single layer is converted into a model which consists of edge and vertices. Edges represent the walls, and the vertices represent the intersections, corners or the end points of wall segments.
Contour Crafting can save considerable time and cost as compared with the traditional way of construction. The cost of construction is related to time and energies spent by the machine and the amount of materials consumed for the structure. The total construction can be evaluated once a tool path has been defined. The building model is first sliced into layers, and then the layout of one single layer is converted into a model which consists of edge and vertices. Edges represent the walls, and the vertices represent the intersections, corners or the end points of wall segments.
A tool path of Contour Crafting for a specific structure must describe the position, orientation, velocity, and deposition rate of the nozzle in the entire construction period. This information is converted into a sequence of machine tasks and then fed to the Contour Crafting machine. If time or energy spent on each machine task (such as deposition, nozzle traveling or nozzle rotation) is defined as cost of construction, then process optimization means finding a path with the minimum total cost associated with every machine task. Therefore, costs of deposition, traveling and rotation need to be defined for calculating the overall cost associated with the tool path. Cost of deposition depends on the flow rate of deposition and the velocity of the machine. However, since the nozzle has to traverse along all the deposition edges once and only once, the overall deposition time is fixed once a structure is given. Therefore, the cost of deposition does not affect the result of tool path optimization. Once the machine parameters have been defined, cost of deposition for each wall segment can be calculated according to its geometrical information. Cost of traveling between edges is related to the cost of moving between vertices and the cost of rotation along the edges. This cost can be estimated according to the relevant position of edges. Each edge has two end points; therefore, there are a total of four possible traveling costs from one edge to another edge. Since the nozzle of the Contour Crafting machine has to orient itself to be perpendicular to the tangent of the wall segment, the nozzle may need to be re-oriented when traveling between edges. For instance, in order to construct a corner, the nozzle must rotate 90° between the constructions of two wall segments. There can be different construction sequences for the nozzle.
Four possible options to construct the corner are shown in this figure. In the top left option the nozzle first builds one wall segment while moving toward the corner.
At the corner the nozzle make a 90° turn and then completes the other wall segment. In the top right option the nozzle starts from the corner, builds one wall segment, diagonally moves to the beginning of the other wall segment while rotating 90° and builds the segment while moving toward the corner. In the lower left option the nozzle starts building one segment while moving toward the corner and then travels to the beginning of the other segment while rotating 90° and starts constructing the segment while moving toward the corner. In the lower right option the nozzle starts at the corner and builds one wall segment while moving away from the corner then travels back to the corner while rotating 90° and then builds the other segment while moving away from the corner. In order to choose the best tool path option the cost of traveling, cost of moving (Euclidian distance) and cost of rotation must be calculated.
Cost of moving between end points can be determined once the distance between two points and the velocity of the machine are known. Sometimes, the nozzle has to be lifted up and lowered down to avoid obstacles, such as previously deposited wall segments. In this case, the cost of lifting up and lowering down also need to be included in the cost of moving. Cost of rotation between edges can be evaluated according to the relative orientation of the two edges. However, the degree of rotation of the nozzle is limited because in the real system there are cables and wires attached to the nozzle to transfer signal and power to move the nozzle components (trowels, valves, vibrators, etc.). If the nozzle is allowed to rotate without limitation, cables and wires may tangle together and get damaged. For this reason, a mechanical stop is installed on the rotation union to prevent the nozzle from turning more than 360° in either direction. Nozzle rotation direction and degree of rotation need to be adjusted if the stopper impedes the re-orientation transition of the nozzle in a given direction. Therefore, the cost of rotation depends not only on the extent of rotation but also on the starting and end positions of the stopper on the rotation union.
Cost of rotation by the same degrees in clockwise and counterclockwise directions may be different due to the limitation that the stopper imposes on the rotation union. Start and end orientations of the nozzle need to be considered in order to find the rotation cost. The rotation cost of arbitrary degrees can be calculated according to the relative start and end positions of the stopper. Once the Euclidian distance and orientation of two edges are known, traveling cost between the points can be estimated by calculating the time spent on moving and rotating. If the nozzle is allowed to rotate while moving from point to point, then cost of traveling between two edges would be equal to the maximum of movement or rotation time, otherwise it will be the sum of the two costs.
2.4. Constraint definitions:
Besides the costs of nozzle deposition and traveling, the following physical constraints also need to be considered during the entire construction process:
1. Nozzle idle time cannot be too long; otherwise concrete may solidify and clog the pipeline. The nozzle idle time cost is equal to the cost of traveling between two wall segments. Therefore, if the cost of traveling between any wall segments is shorter than the time it takes for the concrete in the nozzle to solidify, the requirement of this constraint is fulfilled.
Besides the costs of nozzle deposition and traveling, the following physical constraints also need to be considered during the entire construction process:
1. Nozzle idle time cannot be too long; otherwise concrete may solidify and clog the pipeline. The nozzle idle time cost is equal to the cost of traveling between two wall segments. Therefore, if the cost of traveling between any wall segments is shorter than the time it takes for the concrete in the nozzle to solidify, the requirement of this constraint is fulfilled.
2. The lower layer must be able to support the upper layer, therefore the time interval between depositing subsequent layers cannot be shorter than the critical limit.
3. Subsequent layers must be able to adhere, therefore the interval between depositing subsequent layers should not exceed the critical limit. Constraints 2 and 3 are both related to the time interval between depositing subsequent layers. This interval is equal to the overall time of constructing a layer, which can be calculated once the tool path of the layer has been generated. If the resulting time is shorter than the required interval in constraint 2, the machine has to wait before depositing the next layer. If this time is longer than the required interval in constraint 3, then this indicates that the structure is too large to be built by a single nozzle, and hence more nozzles or gantries would be needed for the construction.
4. When layers accumulate, the underneath layer must be solidified enough to support the overall weight of multiple layers above it. The overall weight on a layer is proportional to the number of layers above it. Hence it is proportional to the overall time of constructing a layer. Once the construction time of each layer is calculated, a chart that shows the relationship between the weight of upper layers and construction time can be used to verify this constraint.
5. Nozzle should not collide with the previously deposited layer when traveling. There are alternative ways to avoid the collision between the nozzle and previously deposited wall segments. When moving between end points of wall segments, the nozzle may have to travel in a non-straight line in order to avoid obstacles. However, although the nozzle can detour to avoid the wall segments, the routing path depends on the structure layout and the sequence of construction. In some cases, the detour may be complicated and may take too much traveling time. Since in this research it is assumed that a nozzle has to complete a layer before starting another layer , the nozzle can be lifted up one layer once it reaches an obstacle (previous wall segment) standing in its travel path. It will be lowered down before it starts to deposit material for a new wall segment. The cost of lifting up and lowering down the nozzle has been considered in tool path planning.
Application in Construction:
Application of CC in building construction is depicted in where a gantry system carrying the nozzle moves on two parallel lanes installed at the construction site. A single house or a colony of houses, each with possibly a different design, may be automatically constructed in a single run. Conventional structures can be built by integrating the CC machine with a support beam picking and positioning arm, and adobe structures, such the ones designed by Cal Earth, may be built without external support elements using shape features such as domes and vaults. Following are some interesting aspects of this automated construction concept:
Application of CC in building construction is depicted in where a gantry system carrying the nozzle moves on two parallel lanes installed at the construction site. A single house or a colony of houses, each with possibly a different design, may be automatically constructed in a single run. Conventional structures can be built by integrating the CC machine with a support beam picking and positioning arm, and adobe structures, such the ones designed by Cal Earth, may be built without external support elements using shape features such as domes and vaults. Following are some interesting aspects of this automated construction concept:
1. Design Flexibility:
The process allows architects to design structures with functional and exotic architectural geometries that are difficult to realize using the current manual construction practice.
The process allows architects to design structures with functional and exotic architectural geometries that are difficult to realize using the current manual construction practice.
2. Multiple Materials:
Various materials for outside surfaces and as fillers between surfaces may be used in CC. Also, multiple materials that chemically react with one another may be fed through the CC nozzle system and mixed in the nozzle barrel immediately before deposition. The quantity of each material may be controlled by computer and correlated to various regions of the geometry of the structure being built. This will make possible the construction of structures that contain varying amounts of different compounds in different regions.
Various materials for outside surfaces and as fillers between surfaces may be used in CC. Also, multiple materials that chemically react with one another may be fed through the CC nozzle system and mixed in the nozzle barrel immediately before deposition. The quantity of each material may be controlled by computer and correlated to various regions of the geometry of the structure being built. This will make possible the construction of structures that contain varying amounts of different compounds in different regions.
3. Utility Conduits:
Utility conduits may be built into the walls of a building structure precisely as dictated by the CAD data. Sample sections made with CC and filled with concrete demonstrate this possibility.
Utility conduits may be built into the walls of a building structure precisely as dictated by the CAD data. Sample sections made with CC and filled with concrete demonstrate this possibility.
4. Paint-Ready Surfaces:
The quality of surface finish in CC is controlled by the trowel surface and is independent of the size of the nozzle orifice. Consequently, various additives such as sand, gravel, reinforcement fiber, and other applicable materials available locally may be mixed and extruded through the CC nozzle. Regardless of the choice of materials, the surface quality in CC is such that no further surface preparation would be needed for painting surfaces. Indeed an automated painting system may be integrated with CC.
The quality of surface finish in CC is controlled by the trowel surface and is independent of the size of the nozzle orifice. Consequently, various additives such as sand, gravel, reinforcement fiber, and other applicable materials available locally may be mixed and extruded through the CC nozzle. Regardless of the choice of materials, the surface quality in CC is such that no further surface preparation would be needed for painting surfaces. Indeed an automated painting system may be integrated with CC.
5. Smart Materials:
Since deposition in CC is controlled by computer, accurate amounts of selected construction materials, such as smart concrete, may be deposited precisely in the intended locations. This way the electric resistance, for example, of a carbon filled concrete may be accurately set as dictated by the design. Elements such as strain sensors, floor and wall heaters can be built into the structure in an integrated and fully automated manner.
Since deposition in CC is controlled by computer, accurate amounts of selected construction materials, such as smart concrete, may be deposited precisely in the intended locations. This way the electric resistance, for example, of a carbon filled concrete may be accurately set as dictated by the design. Elements such as strain sensors, floor and wall heaters can be built into the structure in an integrated and fully automated manner.
6. Automated Reinforcement:
Robotic modular imbedding of steel mesh reinforcement into each layer may be devised.The three simple modular components shown in this figure may be delivered by an automated feeding system that deposits and assembles them between the two rims of each layer of walls built by CC. A three dimensional mesh may be similarly built for columns. Concrete may then be poured after the rims of the wall or column are built by CC. The mesh can follow the geometry of the structure. Note that in this configuration the CC nozzle, the steel reinforcement module feeder, and the concrete filler feeder could all be on the same gantry system. Such a system can create shapes with smooth outer surfaces and reinforced internal structure automatically and in one setup.
Robotic modular imbedding of steel mesh reinforcement into each layer may be devised.The three simple modular components shown in this figure may be delivered by an automated feeding system that deposits and assembles them between the two rims of each layer of walls built by CC. A three dimensional mesh may be similarly built for columns. Concrete may then be poured after the rims of the wall or column are built by CC. The mesh can follow the geometry of the structure. Note that in this configuration the CC nozzle, the steel reinforcement module feeder, and the concrete filler feeder could all be on the same gantry system. Such a system can create shapes with smooth outer surfaces and reinforced internal structure automatically and in one setup.
As an alternative to traditional metal reinforcement, other advanced materials can be used, such as the fiber reinforced plastics (FRP). Since the nozzle orifice in CC does not need to be very small, it is possible to feed glass or carbon fiber tows through the CC nozzle to form continuous reinforcement consolidated with the matrix materials to be deposited. In the proposed study, deposition of the FRP reinforcement by a parallel nozzle built into the CC nozzle assembly will also be considered. Co-extrusion is further discussed in a later section. Reinforcement can also be provided using the post tensioning system. Accurate ducts can be generated by the CC process. Similar to post-tensioned concrete construction, metal or FRP wires can be fed through the ducts and then post-tensioned to provide reinforcement.
7. Automated tiling of floors and walls:
Automated tiling of floors and walls may be integrated by robotically delivering and spreading the material for adhesion of tiles to floors or wall. Another robotic arm can then pick the tiles from a stack and accurately place them over the area treated with the adhesive material. These robotic arms may be installed on the same structure which moves the CC nozzle.
8. Automated plumbing:
Because of its layer by layer fabrication method, a Contour Crafting based construction system has the potential to build utility conduits within walls. This makes automated construction of plumbing and electrical networks possible. For plumbing, after fabrication of several wall layers, a segment of copper (or other material) pipe is attached through the constructed conduit onto the lower segment already installed. The robotics system, delivers the new pipe segment and in case of copper pipes has a heater element (shown in red) in the form of a ring.
The inside (or outside) rim of each pipe segment is pretreated with a layer of solder. The heater ring heats the connection area, melts the solder, and once the alignment is made, bonds the two pipe segments. Other universal passive (requiring no active opening or closing) robotic gripper and heater mechanism designs used form various plumbing components. The needed components may be prearranged in a tray or magazine for easy pick up by the robotic assembly system. Using these components various plumbing networks may be automatically imbedded in the structure.
9. Automated electrical and communication line wiring:
A modular approach similar to industrial bus-bars may be used for automating electrical and communication line wiring in the course of constructing the structure by Contour Crafting. The modules, as shown in Fig.3.7, have conductive segments for power and communication lines imbedded in electrically non-conductive materials such as a polymer, and connect modularly, much like the case of plumbing. All modules are capable of being robotically fed and connected. A simple robotics gripper can perform the task of grabbing the component from a delivery tray or magazine and connecting it to the specified component already installed. The automated construction system could properly position the outside access modules behind the corresponding openings on the walls, as specified by the plan. The only manual part of the process is inserting fixtures through wall openings into the automatically constructed network.
Automated tiling of floors and walls may be integrated by robotically delivering and spreading the material for adhesion of tiles to floors or wall. Another robotic arm can then pick the tiles from a stack and accurately place them over the area treated with the adhesive material. These robotic arms may be installed on the same structure which moves the CC nozzle.
8. Automated plumbing:
Because of its layer by layer fabrication method, a Contour Crafting based construction system has the potential to build utility conduits within walls. This makes automated construction of plumbing and electrical networks possible. For plumbing, after fabrication of several wall layers, a segment of copper (or other material) pipe is attached through the constructed conduit onto the lower segment already installed. The robotics system, delivers the new pipe segment and in case of copper pipes has a heater element (shown in red) in the form of a ring.
The inside (or outside) rim of each pipe segment is pretreated with a layer of solder. The heater ring heats the connection area, melts the solder, and once the alignment is made, bonds the two pipe segments. Other universal passive (requiring no active opening or closing) robotic gripper and heater mechanism designs used form various plumbing components. The needed components may be prearranged in a tray or magazine for easy pick up by the robotic assembly system. Using these components various plumbing networks may be automatically imbedded in the structure.
9. Automated electrical and communication line wiring:
A modular approach similar to industrial bus-bars may be used for automating electrical and communication line wiring in the course of constructing the structure by Contour Crafting. The modules, as shown in Fig.3.7, have conductive segments for power and communication lines imbedded in electrically non-conductive materials such as a polymer, and connect modularly, much like the case of plumbing. All modules are capable of being robotically fed and connected. A simple robotics gripper can perform the task of grabbing the component from a delivery tray or magazine and connecting it to the specified component already installed. The automated construction system could properly position the outside access modules behind the corresponding openings on the walls, as specified by the plan. The only manual part of the process is inserting fixtures through wall openings into the automatically constructed network.
CONCLUSIONS
1. Due to its speed and its ability to use in-situ materials, Contour Crafting has the potential for immediate application in low income housing and emergency shelter construction.
1. Due to its speed and its ability to use in-situ materials, Contour Crafting has the potential for immediate application in low income housing and emergency shelter construction.
2. Construction of luxury structures with exotic architectural designs involving complex curves and other geometries, which are expensive to build using manual approach, is another candidate application domain for CC.
3. The environmental impact of CC is also noteworthy.
4. According to various established statistics the construction industry accounts for a significant amount of various harmful emissions and construction activities generate an exorbitant amount of solid waste.
5. Construction of a typical single-family home generates a waste stream of about 3 to 7 tons. In terms of resource consumption, more than 40% of all raw materials used globally are consumed in the construction machines built for Contour Crafting may be fully electric and hence emission free.
6. Because of its accurate additive fabrication approach Contour Crafting could result in little or no material waste.
7. The CC method will be capable of completing the construction of an entire house in a matter of few hours (e.g., less than two days for a 200 m2 two story building) instead of several months as commonly practiced.
8. This speed of operation results in efficiency of construction logistics and management and hence favorably impacts the transportation system and environment.
9. There are numerous research tasks that need to be undertaken to bring the CC construction technology to commercial use. The activities reported in this article are the first few steps toward realization of actual full scale construction by Contour Crafting.
REFERENCES
1. Jing Zhang , Behrokh Khoshnevis , “Optimal machine operation planning for construction by Contour Crafting”, Automation in construction, Science direct, Vol.29, pp 50-67.
2. Behrokh Khoshnevis Professor, Industrial & Systems Engineering University of Southern California Los Angels, CA 90089-0193,khoshnev@usc.edu,”Automated construction by contour crafting-Related Robotics and Technologies”, Science direct Automation in construction, Vol.13, pp 5-19.
3. Sungwoo Lim*, Richard Buswell, Thanh Le, Rene Wackrow, Simon Austin,
Alistair Gibb, and Tony Thorpe, “Development of viable concrete printing process”.
4. I. Vizotto , “Computational generation of free-form shells in architectural design and civil engineering”, Automation in construction, Science direct, Vol.16, pp 224-231.
5. Danijel Rebolj, Martin Fischer, Drew Endy , Thomas Moore, Andrej Šorgo, “Can we grow buildings? Concepts and requirements for automated nano- to meter-scale building”, Advanced Engineering Informaties, Science direct, Vol.25, pp 390-398
6. R.A. Buswell, R.C. Soar, A.G.F. Gibb, A. Thorpe, “Freeform Construction: Mega-scale Rapid Manufacturing for construction”, Automation in construction, Science direct, Vol.16, pp 224-231.
1. Jing Zhang , Behrokh Khoshnevis , “Optimal machine operation planning for construction by Contour Crafting”, Automation in construction, Science direct, Vol.29, pp 50-67.
2. Behrokh Khoshnevis Professor, Industrial & Systems Engineering University of Southern California Los Angels, CA 90089-0193,khoshnev@usc.edu,”Automated construction by contour crafting-Related Robotics and Technologies”, Science direct Automation in construction, Vol.13, pp 5-19.
3. Sungwoo Lim*, Richard Buswell, Thanh Le, Rene Wackrow, Simon Austin,
Alistair Gibb, and Tony Thorpe, “Development of viable concrete printing process”.
4. I. Vizotto , “Computational generation of free-form shells in architectural design and civil engineering”, Automation in construction, Science direct, Vol.16, pp 224-231.
5. Danijel Rebolj, Martin Fischer, Drew Endy , Thomas Moore, Andrej Šorgo, “Can we grow buildings? Concepts and requirements for automated nano- to meter-scale building”, Advanced Engineering Informaties, Science direct, Vol.25, pp 390-398
6. R.A. Buswell, R.C. Soar, A.G.F. Gibb, A. Thorpe, “Freeform Construction: Mega-scale Rapid Manufacturing for construction”, Automation in construction, Science direct, Vol.16, pp 224-231.
0 comments:
Post a Comment